Redox Reactions & Redox Titrations
Explore the fundamentals of redox reactions, oxidation states, electronegativity, and redox titrations in this short blog.
Reduction-oxidation reactions, or redox reactions, take place all around us. In fact, they’re essential for life on Earth. Redox reactions are the basis of photosynthesis and respiration, processes that benefit all living things. You’ve likely observed the effects of these reactions if you’ve ever picked up an old coin or rusty nail, as the corrosion of metal is a classic redox reaction.
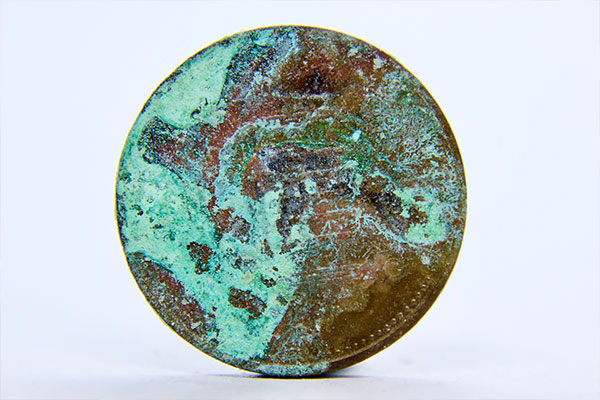
What Happens During a Redox Reaction?
Redox reactions are reactions in which electrons are transferred between atoms, ions, or molecules. One species is oxidized, which means it loses electrons, while another species is reduced, which means it gains electrons. The total number of electrons lost and gained in a redox reaction is always the same.
Oxidation: Loss of electrons | Increase in oxidation state
Reduction: Gain of electrons | Reduction in oxidation state
Let’s consider the reaction that occurs during photosynthesis, in which light energy, carbon dioxide and water produce sugar and oxygen:
6CO2(g) + 6H2O(l) → C6H12O6(s) + 6O2(g)
In the reaction above, carbon dioxide is reduced into sugar (gains electrons), and water is oxidized to oxygen (loses electrons).
Oxidation State
In order to calculate redox reactions, we must first understand the oxidation state—also known as the oxidation number—of the chemical species involved.
The oxidation state (OS) is a measure of the degree of oxidation of an atom in a chemical compound. In other words, it describes the number of electrons an atom loses or gains in order to bond with another atom.
Oxidation states are hypothetical charges we assign to atoms to simplify equations; they’re hypothetical because we are pretending the atoms are behaving as ions (with individual net charges) rather than sharing the electrons covalently. These assigned charges help us understand what is being reduced and what is being oxidized.
Oxidation states are assigned using the following rules:
Element | Oxidation State |
Group 1 Metals | +1 |
Group 2 Metals | +2 |
Oxygen | -2 |
Hydrogen | +1 |
Fluorine | -1 |
Chlorine | -1 |
- The oxidation state of a free, uncombined element is zero.
- The oxidation state of a monoatomic ion (single atom) is equal to the charge of the ion.
- In a polyatomic ion, the oxidation states of the atoms will add up to the overall charge of the ion.
- The sum of the oxidation states of all the atoms in a neutral compound is zero.
The oxidation state of an atom can be positive, negative, or zero. A positive oxidation state indicates that the atom has lost electrons, while a negative oxidation state indicates that the atom has gained electrons. A zero oxidation state indicates that the atom has neither lost nor gained electrons.
Note: When writing oxidation states, it’s a formality to write the positive or negative charge before the number, such as H+1. For a monatomic ion, you may see the oxidation state written in Roman numerals within parenthesis, such as Fe(II) for Fe+2 or Fe(III) for Fe+3 since the charge of the ion equals the oxidation state. For an ionic charge, the charge is written after the number, such as Ca2+.
Let’s consider the chemical process of how iron (Fe) becomes rust, or iron oxide (Fe2O3), below:
4Fe(s) + 3O2(g) → 2Fe2O3(s)
Iron (Fe) goes from an oxidation state of 0 to +3. Oxygen (O) goes from an oxidation state of 0 to -2. Therefore, we know iron is oxidized (loses electrons to become more positive) and oxygen is reduced (gains electrons to become more negative).
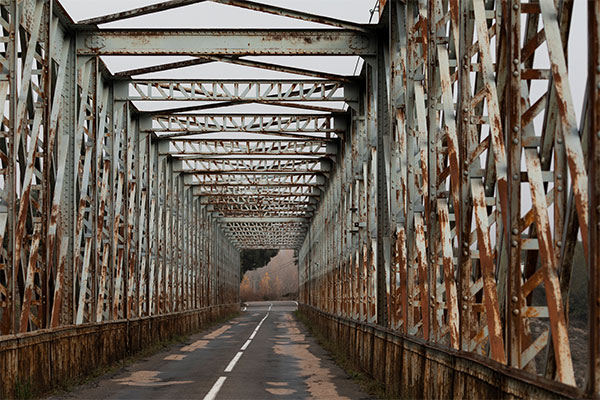
The oxidation state of an atom can help predict its chemical behavior and determine the structure of a compound. For example, atoms with a positive oxidation state are more likely to react with atoms with a negative oxidation state, and vice versa.
Let’s continue with our example of iron. Iron can exist in different oxidation states, including Fe(0), Fe(II), and Fe(III). Fe(III) is more oxidized than Fe(II) (+3 is more positive than +2, indicating it has less electrons) and is therefore more reactive towards reducing agents, or electron-donors. On the other hand, Fe(II) is more easily oxidized than Fe(III) and is thus more reactive towards oxidizing agents.
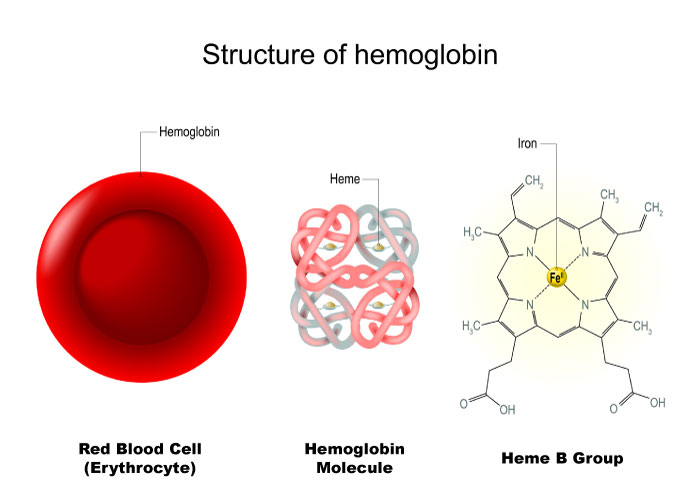
For example, in biological systems, the oxidation state of iron is crucial for its function in redox reactions involved in electron and oxygen transport. In the non-oxygenated hemoglobin of red blood cells, iron is in the Fe(II) oxidation state (ferrous ion), enabling it to bind and transport oxygen; if Fe(II) is oxidized to Fe(III) (ferric ion), hemoglobin no longer accepts more oxygen until the attached oxygen is transferred to surrounding tissues in the capillaries. Similarly, iron is utilized as an electron donor and acceptor in the electron transport chain (ETC).
Electronegativity
Whether atoms are good oxidizing or reducing agents depends on their electronegativity. More electronegative atoms, like oxygen and fluorine, have a stronger affinity to pull electrons away from other atoms causing the atoms they interact with to have higher oxidation numbers. Atoms that are strongly electronegative are good oxidizing agents.
The periodic table below has an arrow showing the direction electronegativity increases across the table.
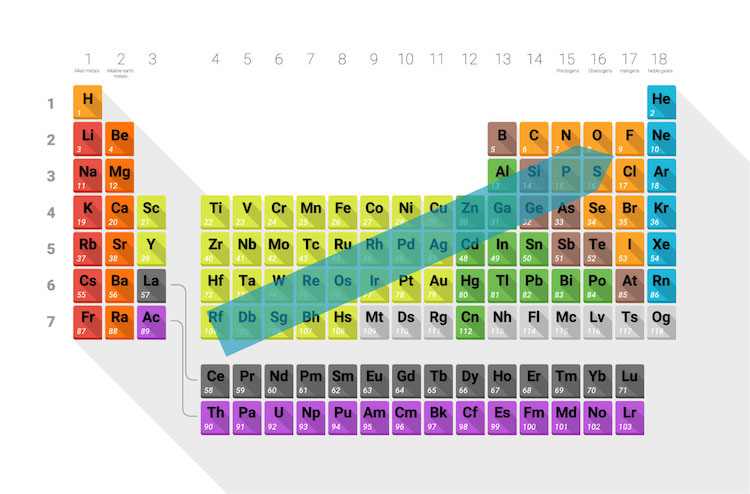
Single-Replacement Reaction
While there are many different types of redox reactions, many can be classified as a single-replacement reaction. In a single-replacement reaction, one element replaces another in a compound.
The general formula for a single-replacement reaction is shown below:
A + BC → AC + B
An example of a single-replacement reaction in the natural world is the reaction between iron and copper sulfate solution. Iron is more reactive than copper, so when a piece of iron is placed in a solution of copper sulfate, the iron will replace the copper in the solution, forming iron sulfate and copper metal. The equation below shows the reaction between iron and copper sulfate. The iron is oxidized and the copper is reduced:
Fe + CuSO4 = FeSO4 + Cu
Rust forms when iron reacts with oxygen and water to form iron oxide (rust) and hydrogen ions. In the presence of copper ions, the reaction proceeds faster due to the single-replacement reaction between iron and copper ions. As a result, copper-colored rust appears on iron pipes that are exposed to moisture and air.
Redox Titrations
During a redox titration, the experimenter uses a burette to titrate a known concentration of solution (titrant) to an unknown concentration of solution (analyte). The procedure may involve the use of one or more of the following: a redox indicator, potentiometer, or an oxidation reduction probe (ORP).
The process of a redox titration can be divided into the following steps:
- Preparation of the analyte solution. Prepare the analyte solution by dissolving a known amount of the analyte in a suitable solvent.
- Preparation of the titrant solution. Prepare the titrant solution by dissolving a known amount of the titrant in a suitable solvent.
- Selection of the indicator. The indicator is a substance that changes color in the presence of the titrant. Select the indicator so that it changes color at the equivalence point of the titration.
- Titration of the analyte solution. Titrate the analyte solution with the titrant solution until the indicator changes color. This point is called the endpoint of the titration.
- Calculation of the concentration of the analyte. Calculate the concentration of the analyte by using the following equation:
Redox titrations are used to find the concentration of many analytes, including metals, ions, and organic compounds, as well as determining the purity of a substance.
Teaching Tools: Oxidation Reduction Potential Probe
Monitor solutions during redox reactions with the Oxidation Reduction Potential Probe! Conduct studies in water quality, corrosion, respiration and photosynthesis, and more. The probe comes with a 2m cable and connects to the Wireless pH sensor to determine whether the species in solution is an oxidizing or reducing agent.

Learn More |
For example, we can determine the concentration of iodine (I2) in a solution by titrating it with a solution of sodium thiosulfate (2S2O32-). The reaction is as follows:
I2 + 2S2O32- → 2I- + S4O62-
Iodine is reduced to iodide ions, and sodium thiosulfate is oxidized to tetrathionate. You can detect the endpoint of the titration by adding a starch indicator, which turns a deep blue color in the presence of iodine. Then, continue the titration until the blue color fades, which indicates that all of the iodine has reacted with the sodium thiosulfate.
The concentration of iodine can be calculated using the following equation:

Redox reactions are a fundamental part of chemistry, and they are used in many different chemical processes and industries. Learning about redox reactions can help you understand the chemistry that surrounds you on a daily basis.